In the human body, adenosine triphosphate (ATP) is the primary energy currency for humans cells and also all living organisms from fungi to bacteria to plants and animals. Understanding the intricate role of ATP in cellular processes is crucial for comprehending the intricate mechanisms that drive life at a microscopic level.
ATP is classified as a nucleic acid and is composed of three fundamental components: adenine, a nitrogenous base; a five-carbon ribose sugar; and a triphosphate chain containing three phosphate groups.
The phosphate groups in ATP, known as alpha, beta, and gamma from closest to farthest from the ribose sugar, are linked by high-energy phosphoanhydride bonds.
Its structure, with high-energy phosphate bonds, allows for the storage and release of energy, which is the driving force behind the functionality of ATP. Upon the cleavage of these bonds, a surge of energy is released, propelling a myriad of cellular functions essential for the sustenance of living organisms.
When these bonds are broken, the release of energy fuels a myriad of cellular functions, enabling processes such as muscle contraction, nerve impulse transmission, and biosynthesis to occur efficiently.
Phosphates are complex compounds fundamental to all cells and help form and repair our DNA and RNA. Phosphate is an anion composed of phosphorous (P) and oxygen (O) atoms.
Phosphorous is an element whereas phosphate is an anion.
An anion is an ion with negative charge, meaning it has more electrons than protons.
It is important that we understand that all life on earth shares the elemental energy source of phosphorous. It is derived from Ancient Greek φωσφόρος (phōsphóros, “the bearer of light”), from φῶς (phôs, “light”) + φέρω (phérō, “to bear, carry”). In Latin, it is known as lucifer and is encoded in Scripture in the Book of Issah as “fallen from heavan.”
When a cell requires energy to carry out essential processes such as muscle contraction, active transport of molecules across membranes, or synthesis of macromolecules, it initiates the breakdown of ATP. Enzymes within the cell catalyze the hydrolysis of ATP by breaking the bond between the terminal phosphate group and the rest of the molecule.
This process results in the formation of adenosine diphosphate (ADP) and inorganic phosphate (Pi), along with the release of energy that was stored in the phosphoanhydride bond. The energy released powers the specific cellular activity that required it, allowing cells to perform tasks vital for their survival and function.
ATP is also known as a purine, which means it is one of two chemical compounds that cells use to make the building blocks of DNA and RNA. They are found in mainly in meat and meat products and are broken down by the body to form uric acid, which is passed in the urine.
Purines such as ATP and adenosine play a central role in the energy metabolism of all life forms. They are released from neurons and glial cells which produce widespread effects on multiple organ systems by binding to purinergic receptors located on the cell surface.
ATP is essential for various cellular processes, including brain function, muscle contraction, biosynthesis, and active transport processes in cells. Active transport involves the movement of molecules or ions against their concentration gradient, requiring energy input. It acts as an excitatory neurotransmitter in motor neurons of the spinal cord, as well as sensory and autonomic ganglia.
Through the cleavage of these phosphoanhydride bonds, ATP releases the energy needed to drive processes such as muscle contraction, nerve impulse transmission, protein synthesis, and various biochemical reactions within cells. This energy transfer mechanism not only sustains basic cellular functions but also enables organisms to grow, reproduce, and respond to environmental stimuli.
When this system is altered or is not getting and or recycyling an adequate amount of ATP through diet and excercise, then these processes become corrupt causing orgamisms such as humans to stop growing and able to respond adequately to environmental stimuli.
I contend that you physical and mental capacities enter into an automanous or sleep like state.
HOW DO WE CREATE ATP?
ATP is created when we eat food. Especially meat and dairy products.
When food is consumed, it undergoes digestion by our microbiome, i.e.: fungi and bacteria which we feed to break down our food into vitamins and ATP energy.
Without this community of microbes that lives symbiotically within and around us, we would not be able to break down the food we eat. Hence, we would simply not exist.
My contention is that this relationship can be compared to a master and slave being that we are slaves and they are our masters.
This is why they can become paraistic when we do not give these microbes the nutrients they require. As if there is an automantonus kill switch withit our cells that turns on when we digress againts these natursal laws. Instead of eating the good food we supply them with, they will eat us and the science proves this.
Let me explain how this happens…
ATP synthesis is the process by which ATP is produced, typically occurring in the mitochondria of eukaryotic cells through cellular respiration by our microbiome. During this process, energy is generated and stored in the form of ATP, ready to be used for various cellular functions.
With that said, it is not you and I who create this energy, but the fungi and bacteria within our guts.
This microbial energy is created by what scientists call, “ATP synthase.”
This means our microbiome rotates in a clockwise manner (like hydroelectric turbines) in response to eating food causing proton flow coupled with ATP synthesis to catalyze the conversion of Adenosine diphosphate (ADP) to ATP.
Here is a computer animation of this process that occurs within a “healthy human gastrointestinal tract.”
This quote from a team of scientists from Germany and Japan to summarize their study of ATP synthase:
“ATP synthase is an iconic intelligently designed molecular machine because of its rotary engine and its universal distribution. Similar forms of these rotating machines, one-tenth the size of the famous bacterial flagellum, are found in all animal cells, plant cells, bacteria, and archaea.
The precision machine raises a huge challenge to all origin-of-life theories: how could a working cell arise without these irreducibly complex molecular machines?
They are vital to every organism on earth.
I heard a biologist at JPL say that if they stopped working, we would be dead before we hit the floor.
For eukaryotic ATP synthase, there is a rotor and stator in the FO domain that turns a “crankshaft” to operate the F1 domain. In the F1 domain, three pairs of catalytic units combine ADP (adenosine diphosphate) with phosphate to produce ATP (adenosine triphosphate), the universal energy currency for most cellular processes.
A main purpose of the food we eat is to create a flow of ions to operate these machines.
ATP synthase is the last machine in a series of complexes in our mitochondria whose collective function is to donate electrons to various intermediates so that protons can be extracted. Protons accumulate between the mitochondrial membranes and flow into ATP synthases.
The machines are lined up in pairs along the folds of the mitochondria (cristae) to take advantage of the proton motive force. That flow of protons turns the FO domains like waterwheels, generating three ATP per revolution in the F1 domains, as shown in the animation.”
ADP can be converted back to ATP through a process called phosphorylation, in which a phosphate group is added back to the molecule using energy from other cellular processes such as respiration or photosynthesis.
Phosphorylation is the process by which protons move through the ATP synthase releasing energy that causes the rotor and rod of the ATP synthase to rotate. The mechanical energy from this rotation is converted into chemical energy as phosphate is added to ADP to form ATP.
The release of one or two phosphate groups from ATP, a process called dephosphorylation, releases energy, i.e., the removal of a phosphate group from an organic compound changing ATP to ADP.
Respiration is the act of respiring, which is the process by which a living organism or cell takes (inhales) oxygen from the air or water and exhales it. Photosynthesis is the process by which animals and plants use sunlight, water, and carbon dioxide to create oxygen and energy in the form of sugar.
This is exactly why today many health practitioners and coaches highly recommend people breathe properly to get proper oxygen intake and go out into the sun as often as possible.
The main purpose is to keep our cells oscillating/rotating in a clockwise manner with the clock of the earth (circadian rhythms) through environmental cues and managing bodily processes by consciously being aware and controlling them to a certain degree.
It is interesting to note that our microbiome creates and is in control of these natural rotations or oscillations in our gastrointestinal tracts.
I contend that this rotation does not only occur within our GI tracts, but also around our bodies as a type of signaling molecule for these same said microbes.
Scientisists call this the “purine signaling pathway.”
A purine is an aromatic ring of atoms composed of carbon and nitrogen. The main purinergic receptors are adenosine, ATP and UTP and purines include adenine and guanine, which participate in DNA and RNA formation
Purines are aromatic which means they have an aroma or smell.
This is why the human microbiome, comprising trillions of microorganisms residing in our gut, skin, and other body surfaces, plays a crucial role in not only maintaining our overall health and well-being, but also the way we smell and our bodily wastes smell.
This smell, I theorize, has a certain wavelength that signals or magnetizes these microorganisms to either live symbiotically within and around us or to command them to parasite/eat.
This is why when our ATP intake is low, fungal overgrowth will start to occur within our bodies.
This “purine signal” I theorize acts like a magnet or computer program that sends out wavelengths based on its energy production or lack thereof. Since fungi do not have eyes, ears and a nose, these wavelengths serve as biological commands in our environments for these microorganisms such as the fungi Candida and Aspergillus to either live mutually or symbiotically or for this relationship to become parasitic.
As within, so without and as without, so within.
According to a recent study:
The skin is colonized by a diverse microbiota. Many cutaneous organisms produce molecules that regulate colonization by other microorganisms and modify their intrinsic biology and behavior. The community of commensal species can act synergistically to alter local immune reactivity for mutual benefits of the commensals and the host without leading to pathology.
As part of a dynamic equilibrium between proinflammatory and immunoregulatory signals, extracellular adenosine (ADO) triphosphate (eATP) produced by commensal flora plays an important role in the regulation of immune detection, immune response, and ultimately a balance between host and commensal organisms such as Candida albicans (Mascanfroni et al., 2015).
The term “glia,” originating from the Greek word for “glue” because it is our glia cells that act as a type of super glue or more appropriately a carbon film like matrix that create an interface with oustide stimuli and organisms.
Glial cells play pivotal roles in the intricate functioning of the brain. Beyond their traditional perception as mere “glue” cells, glial cells play a crucial role in modulating the speed and efficiency of nerve signal transmission in maintaining the brain’s homeostasis and functionality.
One of the key functions of glial cells is their role in regulating the ionic environment surrounding nerve cells. By maintaining precise levels of ions such as potassium and calcium, glial cells create an optimal milieu for efficient signal propagation. This fine-tuned control helps ensure the rapid and accurate transmission of electrical impulses along neural circuits, ultimately contributing to the proper functioning of the brain.
Moreover, glial cells contribute significantly to the speed and efficiency of nerve signal transmission playing a crucial role in modulating synaptic activity by regulating the uptake of neurotransmitters. This function allows for precise control of neuronal communication, impacting various cognitive processes and behaviors.
Astrocytes, a prominent subtype of glial cells, play a crucial role in synaptogenesis and synaptic pruning, processes essential for refining neuronal connectivity and optimizing neural circuitry. By regulating neurotransmitter levels and providing metabolic support to neurons, astrocytes fine-tune synaptic transmission and facilitate efficient neuronal communication.
In 1994, researchers conducted experiments where they stimulated astrocytes in a dish, observing nearby neurons preparing to send signals in response. Building upon this, in 1997, Volterra and his team observed rat astrocytes responding to neurons with oscillating waves of the signaling molecule calcium.
Over the span of 2000 to 2012, more than 100 papers were published supporting the notion of astrocytes communicating via synapses.
While neurons are commonly depicted as trees with branching dendrites, astrocytes resemble a fungus, creating a dense network that covers the brain and facilitates information exchange among its components.
This intricate web of astrocytes appears to play a role in influencing neuronal activity. Further research unveiled fascinating insights into astrocytes’ impact on brain function.
For instance, in 2016, Kira Poskanzer’s work at the University of California, San Francisco revealed that mouse astrocytes can induce neighboring neurons into a rhythmic sleep state, highlighting the dynamic interplay between astrocytes and neurons in shaping brain activity.
According to UCLA:
Astrocytes have been implicated in a range of neurological and psychiatric disorders, including Alzheimer’s disease, Parkinson’s disease, epilepsy, stroke, traumatic brain injury and autism. Certain aspect of astrocyte function helps neural repair whereas astrocyte dysfunction exacerbates diseases. Scientists are only beginning to understand what roles astrocytes play in each type of brain disorder.”
Astrocytes are adept at controlling the concentration of ions in the extracellular space, which is essential for maintaining the resting membrane potential of neurons. By regulating the levels of ions, glial cells help in preventing excessive neuronal excitability or inhibition, thus contributing to the stability of neural signaling.
The discovery of astrocytes in 1865 marked a turning point, leading scientists to delve deeper into the world of glial cells and uncover a vast array of functions that go beyond mere structural support. From regulating the ionic environment surrounding nerve cells to influencing nerve signal transmission speed, controlling neurotransmitter uptake, and aiding in neural development through scaffold provision, glial cells have proven to be indispensable players in the neural orchestra.
This newfound understanding challenges the traditional notion of glial cells as passive bystanders and underscores their active and crucial involvement in brain physiology.
CONTROL YOUR GUT (MICROBIOME) OR IT WILL CONTROL YOU!
Our microbiome influences our digestion, metabolism, immune system, and even mental health. It aids in the breakdown of food, the synthesis of essential nutrients, and the protection against harmful pathogens.
These tiny inhabitants consist of bacteria, viruses, fungi, and other microorganisms that form a complex ecosystem within and AROUND us.
This intricate network of microbes communicates with our cells and influences gene expression, demonstrating its profound impact on our physiology.
This symbiotic and sometimes parasitic relationship between our microbiome and energy production pathways underscores the interconnectedness of our body’s systems and the profound impact it can have on our overall well-being.
Meaning exercise and movement, which causes our cells to respire and going the sun are important for this process to work correctly.
Regulation and Recycling of ATP in Living Organisms
Regulation and recycling of ATP in living organisms are vital processes that ensure the continuous availability of energy necessary for cellular activities. The intricate balance of ATP levels within cells is tightly regulated to meet the dynamic energy demands of various biological processes.
Cells possess sophisticated mechanisms to maintain optimal ATP levels. One crucial aspect of ATP regulation involves the enzymes responsible for ATP synthesis and degradation. ATP synthase, a key enzyme in cellular respiration, facilitates the production of ATP from adenosine diphosphate (ADP) and inorganic phosphate (Pi) during the process of oxidative phosphorylation.
Conversely, ATPases are enzymes that catalyze the hydrolysis of ATP to ADP and Pi, releasing energy that fuels cellular processes. ATP Hydrolysis is a chemical reaction where a phosphate bond that has been stored within ATP is broken by water after splitting these bonds, for example in muscles, by producing work in the form of mechanical energy.
When it comes to ATP production, understanding the differences between aerobic and anaerobic respiration pathways is crucial.
Aerobic respiration is the process that occurs in the presence of oxygen and is highly efficient, producing a large amount of ATP from glucose molecules. This process takes place in the mitochondria of cells and involves a series of complex reactions, including the citric acid cycle and oxidative phosphorylation.
On the other hand, anaerobic respiration occurs in the absence of oxygen and is less efficient compared to aerobic respiration. One common example of anaerobic respiration is fermentation, where glucose is partially broken down to produce ATP and byproducts such as lactic acid or ethanol fermentation. I
While anaerobic respiration can provide a quick burst of energy, it is not sustainable for long periods due to the accumulation of lactic acid which can lead to muscle fatigue.
ATP’s function is entirely reliant on the availability of ADP. Without an adequate supply, ATP synthase ceases to function correctly, causing it to dysfunction and decreased ATP production.
Meaning, at the cellular level, your cells start to stop spinning and producing energy.
In fact, it can start spinning counter clockwise.
This then rewires energy metabolism and causes enhanced glycolysis and inflammatory processes, which are a common feature of many age‐associated diseases, including Alzheimer’s, muscular dystrophy, ALS, diabetes and cancer.
This is called microbiota dysbiosis, which refers to an imbalance in the composition and function of the microbial communities that reside in our gut.
The meaning of the compound word dysbiosis is from the Greek dys which means bad and biosis, the way of life.
Dysbiosis has been linked to conditions such as irritable bowel syndrome (IBS), inflammatory bowel disease (IBD), small intestinal bacterial overgrowth (SIBO), depression, anxiety, and autism spectrum disorders.
Intestinal epithelial cells (IECs) sense microbial stimuli through a number of different mechanisms that regulate IEC gene transcription and inflammatory responses.
It plays an important role in the digestion of food, absorption of nutrients, and protection of the human body from microbial infections, and others.
The intestinal epithelium is a highly dynamic tissue that provides both physical and chemical barriers to protect the intestinal mucosa and peripheral organs from commensal microbes or invading pathogenic microorganisms. In addition to forming a barrier, IECs also detect a myriad of signals from intestinal microbes, allowing fine tuning of IEC proliferation and homeostatic functions
It is the interface between us and them.
A deficiency in ATP synthase, believed to be triggered by mutations in the mtDNA genes for ATP subunits, contributes to various genetic mitochondrial diseases. Moreover, a range of conditions, including Alzheimer’s, muscular dystrophy, ALS, diabetes, and cancer, can induce secondary mitochondrial dysfunction.
Unfortunately, there are currently no known treatments for either secondary ATP deficiency or genetic mitochondrial diseases. However, researchers at the University of Colorado (CU) have been delving into the symbiotic relationship between gut bacteria and the human body, potentially uncovering a breakthrough for addressing ATP synthase deficiency.
They have devised a method to circumvent this limitation by leveraging fast-growing filamentous fungi, Neurospora crassa.
Neurospora crassa is a type of red bread mold of the genus of Ascomycete. The name, meaning ‘nerve spore’ in Greek, refers to resemblance to brain axons.
Neurospora research has found this fungi to be especially useful for studies of photobiology, circadian rhythms, population biology, morphogenesis, mitochondrial import, DNA repair and recombination, DNA methylation, and other epigenetic processes (Borkovich et al. 2004).
To be continued…
SOURCES:
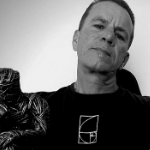
Moe is the founder of GnosticWarrior.com. He is a father, husband, author, martial arts black belt, and an expert in Gnosticism, the occult, and esotericism.